System-level approach to NVH models
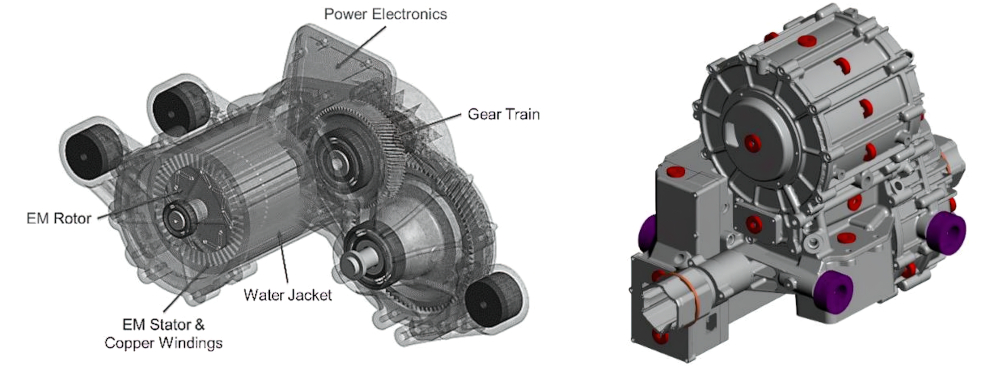
Complex interactions between electric powertrain components provide significant challenges in accurately predicting the noise, vibration and harshness (NVH) performance of a design when using high level system-level models (writes Nick Flaherty).
The models can help reduce the cost and development time, but the underlying interaction of the component-level simulations needs to be understood to achieve the necessary accuracy.
To do this, engineers at Drive System Design (DSD) tested out various motor models, looking at the stator orthotropic material properties, the influence of windings and damping factors. These findings were used in an assembled motor test model and correlated with housing accelerometer response test data to map the system models to real-world data.
The noise issues commonly seen in EVs arise mostly from the complex interactions between components. However, as hardware is typically not available in the early stages of a design and is costly to test, simulations are used instead, and their models and methods have to represent real-world components and be able to accurately predict NVH issues.
Electric drive units (EDUs) have shared structures between the motor, transmission and inverter, which each generate excitation sources, so a system-level approach to the simulation is required. Including these key components in a single model, usually up to the isolation mounts, enables these interactions to be captured, and this complex behaviour would otherwise be missed if individual component models were analysed.
A typical system NVH model includes finite element (FE) models of complex geometries such as the housing, differential, stator and complex gear blanks. Non-linear stiffness models should be included to represent bearings and mount locations.
The effect of the excitations and the transfer path to outer casing is simulated using a combination of FE and 1D representations where appropriate. This results in a response at the casing, usually in the form of virtual accelerometers or radiated noise.
Stator lamination stacks for example can be challenging to model. They are made from many bonded laminations and unlike many solid mechanical components, which can be modelled as an isotropic structure, behave orthotropically and must be modelled in a different way.
This can have a major effect on the response of the motor, and in turn the EDU. The complex damping characteristics of the stator, in part owing to the bonding process and materials, are important to capture in order to correctly determine the magnitude of response.
Motor windings add significant mass to the stator sub-assembly, and the data for this must also be captured. However, the effect on the stiffness of the stator teeth, and the influence on damping, are also important characteristics in determining the overall response.
The rotor has similar challenges, and this can influence the stiffness of the transfer path from the excitations on the rotor into the transmission structures.
The motor winding, rotor and stator all have multiple harmonics and considerations to capture, such as motor torque ripple and stator tooth forces in the radial, tangential and axial direction for the motor.
The influence from the inverter, such as pulse width modulation on the motor current ripple, also needs to be captured to ensure accurate motor excitations.
There are also excitations due to manufacturing deviations, such as rotor imbalance excitations, which is becoming even more important with the trend towards higher speed motors. All these excitations must be considered together against the full-system structural model.
Component-level correlation aids the development of system simulation models, and also improves general processes and understanding. This can allow correlation from one design to increase confidence for future analysis prior to producing hardware.
DSD’s engineers point out there are still many challenges in the optimisation of NVH performance in EVs. Advances in FE techniques, particularly combining methods such as representing transient contact conditions and integrating them into system NVH models, will enable the next phase of complex development in EVs. Sound quality targets and psychoacoustic metrics are quickly advancing, and using simulation to aid sound quality target definition and verification will benefit system developers.
ONLINE PARTNERS
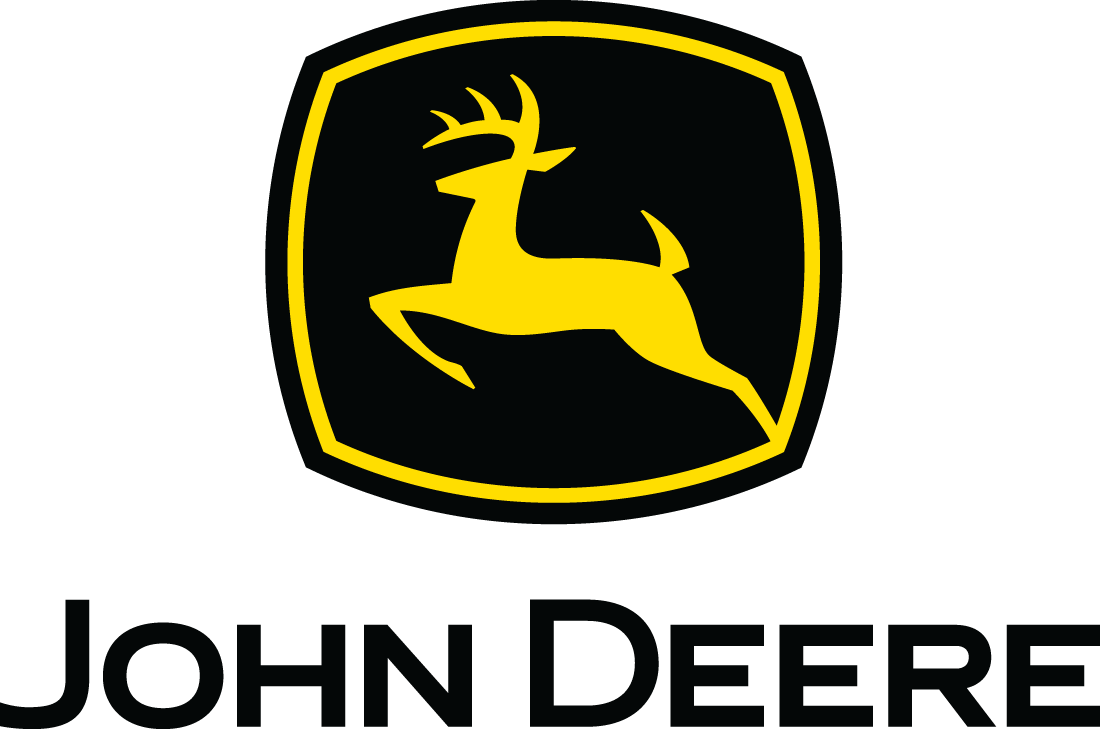
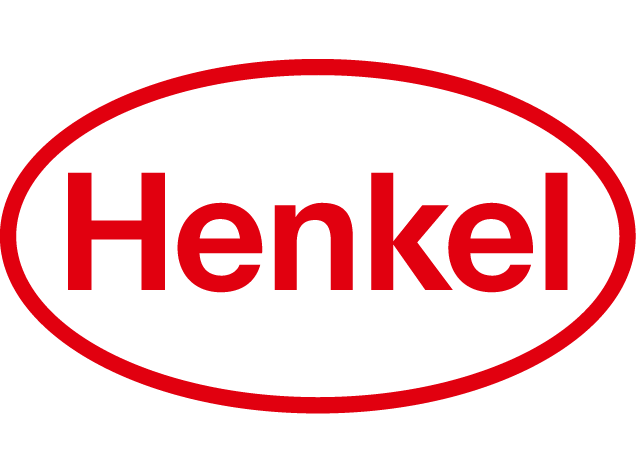
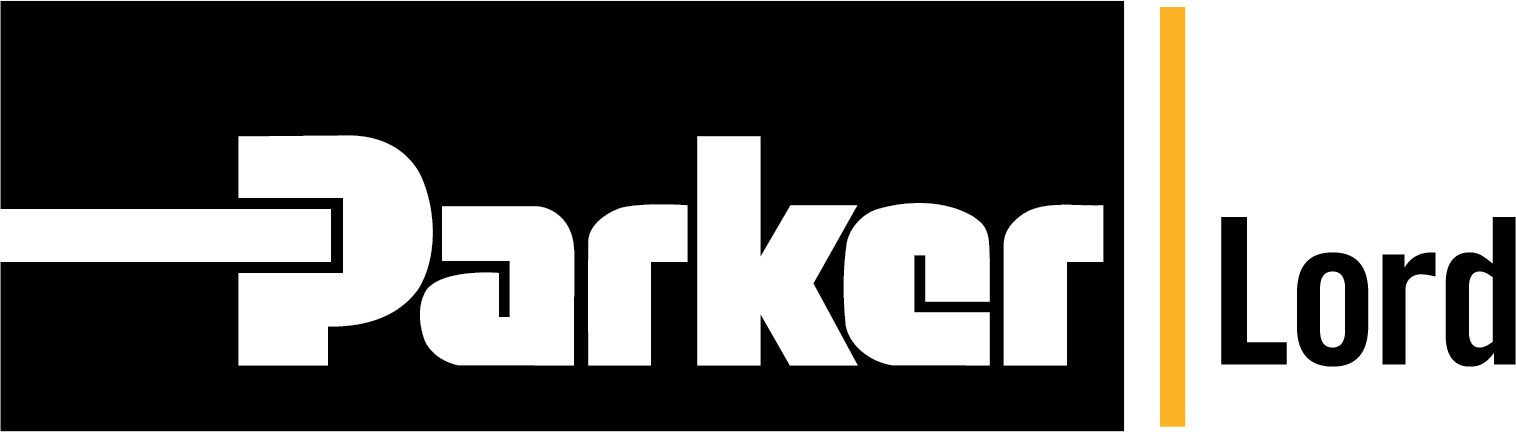
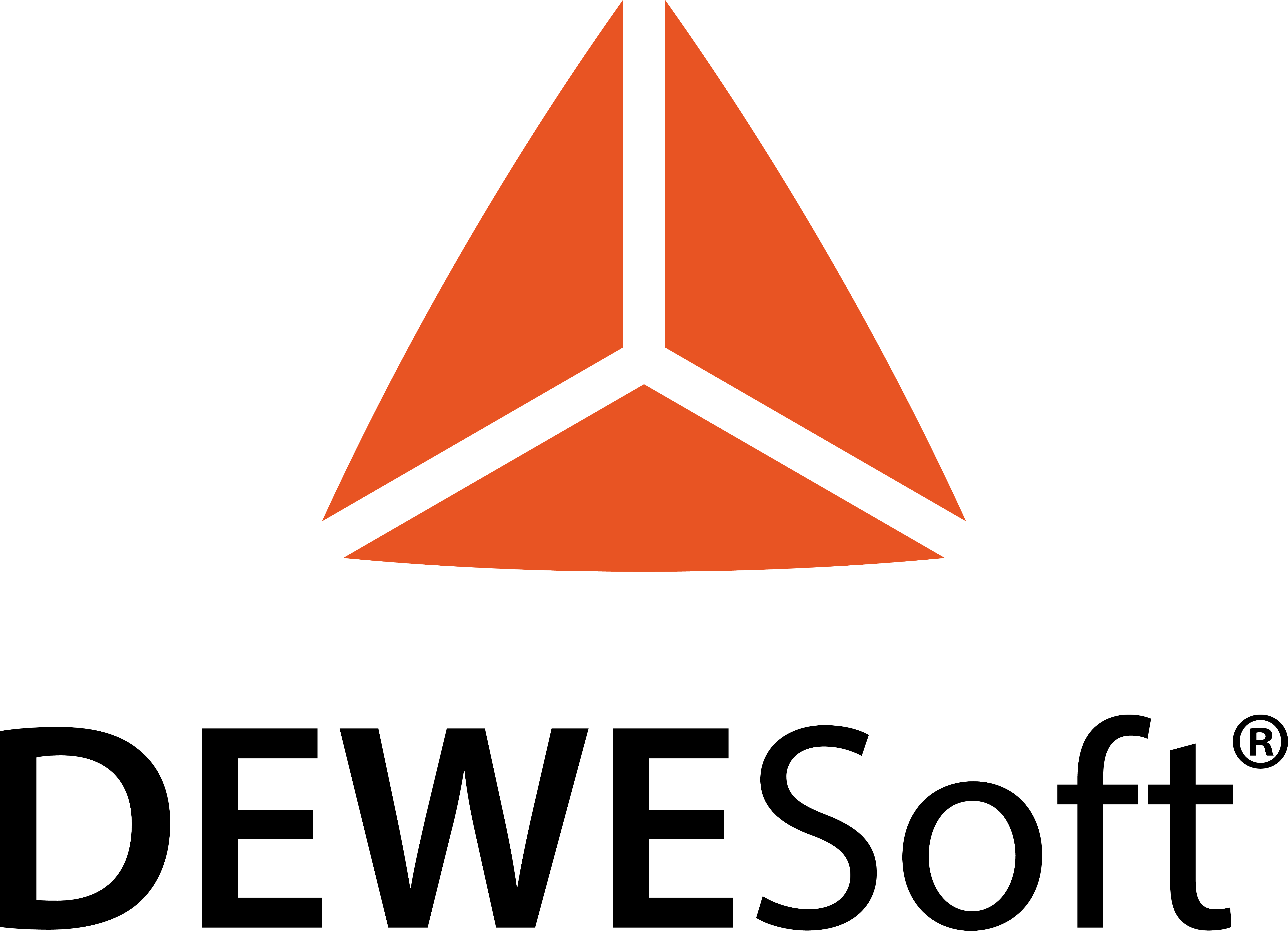
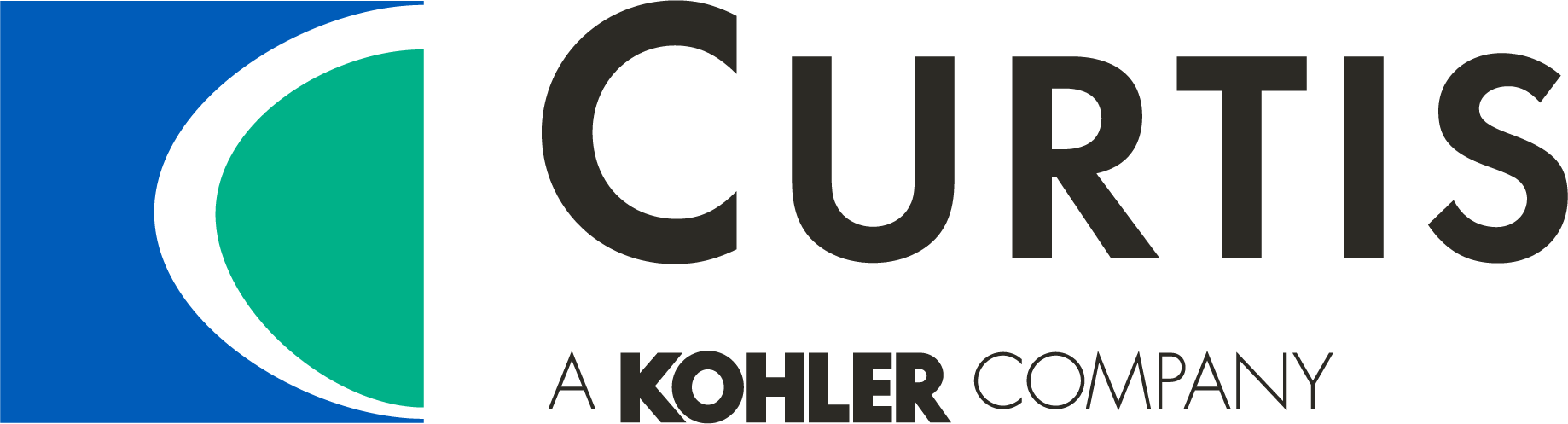
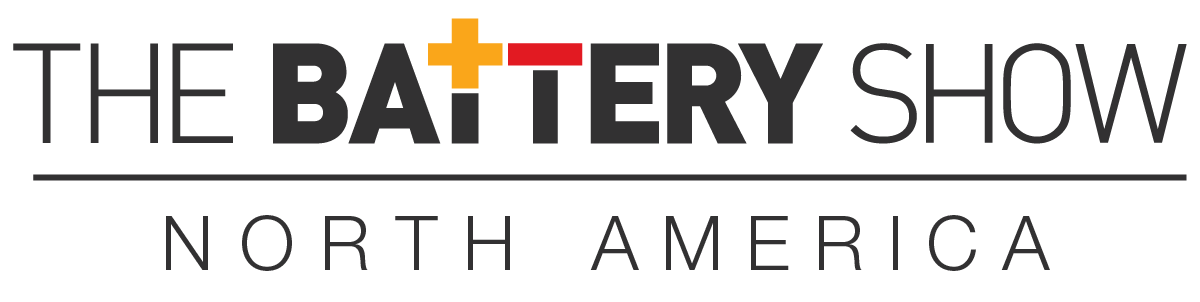