Researchers gain insights into improving lithium-ion cells
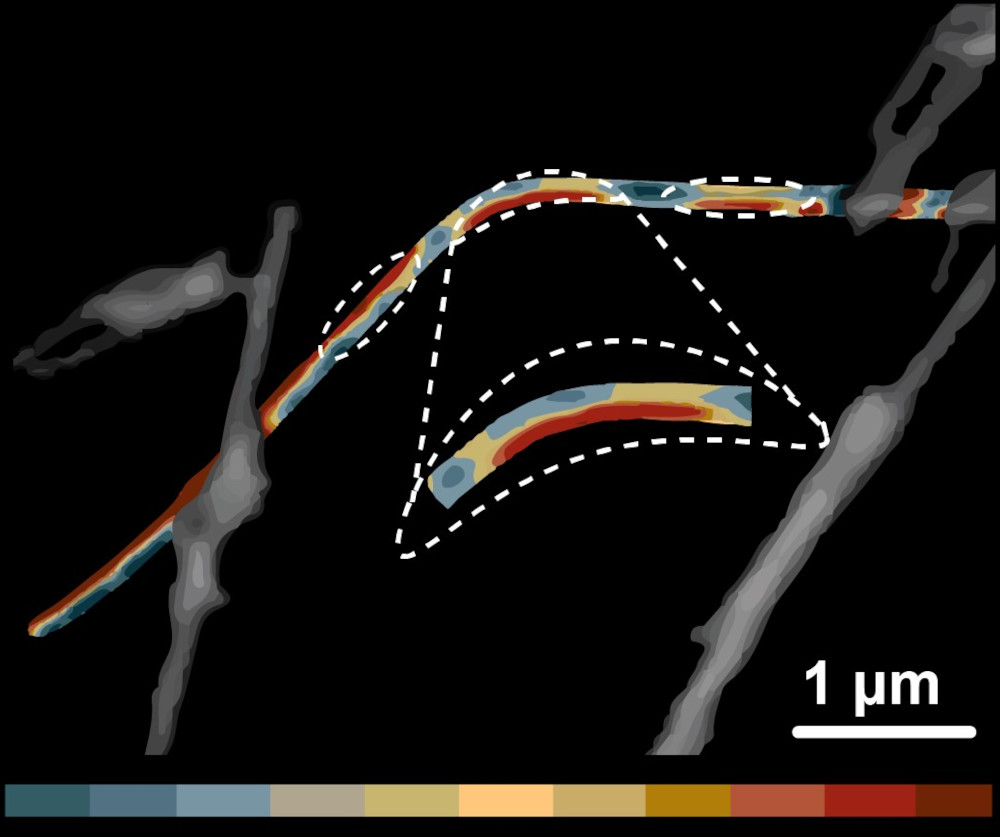
(Courtesy of Texas A&M University)
A team of scientists from the US, Canada and Germany (led by researchers at Texas A&M University, TAMU) have combined novel imaging techniques and large data sets to try to gain a better understanding of why lithium-ion energy storage systems fail, and hence how they can be improved (writes Rory Jackson).
A key focal point of the research group was to better understand what happens in lithium-ion batteries at the nanoscale level, with particular regard to the stress gradients and subsequent degenerative failures originating from the use of heterogeneous battery electrode materials. Such failures not only reduce the lifespan of battery cells and modules, they also have negative impacts on a battery’s performance over its lifetime and increase the incidence of safety hazards.
To study and measure these stress gradients at the nanoscale, the group worked closely with Canadian Light Source (CLS), a national research facility of the University of Saskatchewan. Using the SM (spectromicroscopy) beamline at CLS, the team was able to visualise the flaws and map defects that occur within the batteries.
“The SM beamline uses intense X-rays to image materials with nanometre-scale spatial resolution,” Sarbajit Banerjee, Professor of Materials Science & Engineering (and Chemistry) at TAMU explained.
“The beamline was used to reconstruct composition and stress maps of single particles and ensembles of particles, thereby yielding a close-up view of charge and discharge mechanisms.
“The measurements enabled observation of some key origins of battery failure. Specifically, high-resolution X-ray images showed us how lithium ions are concentrated within individual particles, and across networks of particles, and the consequences therein for accumulation of stress in the system.”
Notably, the experiment looked into lithium vanadium oxide (LiV2O5) batteries, rather than the more commercially well-known cathode chemistries such as NMC or LFP. When queried about this, Prof Banerjee replied, “Vanadium oxide cathodes are attracting increasing positive attention, given the geographically dispersed supply chains and lack of criticality issues for vanadium.
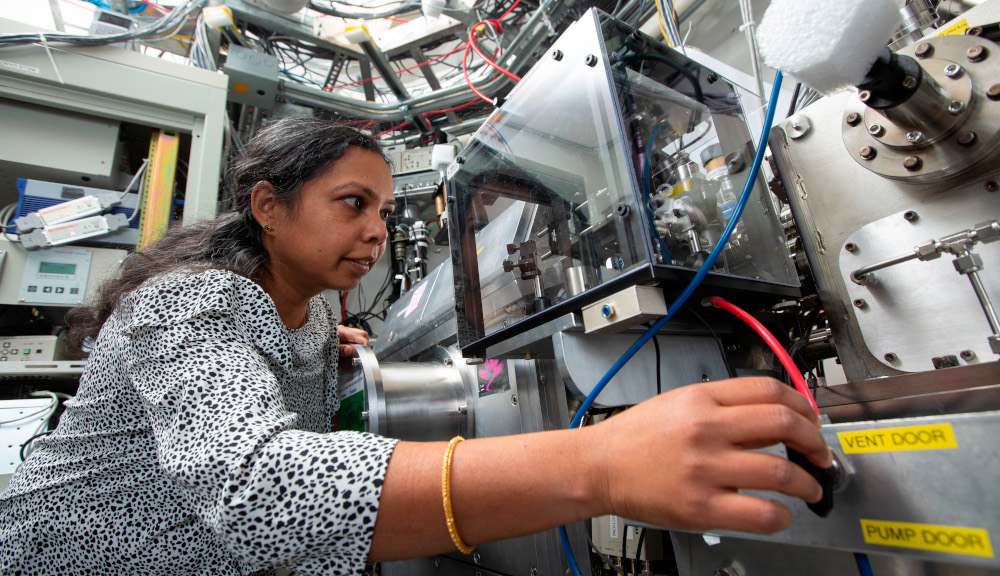
(Courtesy of Canadian Light Source)
“These issues have emerged as a major concern for cobalt chemistries. Furthermore, vanadium battery chemistries allow for multi-electron redox, and show excellent rate performance and cyclability. Several start-ups are making rapid advances in this space, and the emerging landscape of vanadium resources coming online across the world is truly unprecedented.”
The research is therefore also a significant enabler for LiV2O5 as a potential next-generation battery for EVs and other systems. Despite its present-day availability and use as a cathode material for small-format commercial cells, LiV2O5 solutions to date have been prone to a series of intercalation-induced phase transformations. These give rise to stress and diffusion impediments, and limit the accessible energy density and cycle life.
As a result of being able to image lithium-ion battery stress concentrators down to single particles, and hence observing some of the challenges with fully using the capacity of the LiV2O5 cathode, the team successfully imaged the stress accumulated within its particles and across its networks of particles responsible for driving damage and loss of capacity in batteries.
With such stress often driving damage and loss of capacity in LiV2O5 and other cells, ongoing work by the researchers is focused on coming up with a manifesto of design strategies that will mitigate – or even entirely prevent or undo – stress accumulation in intercalation batteries, paving the way for robust and long-lifetime energy storage systems.
ONLINE PARTNERS
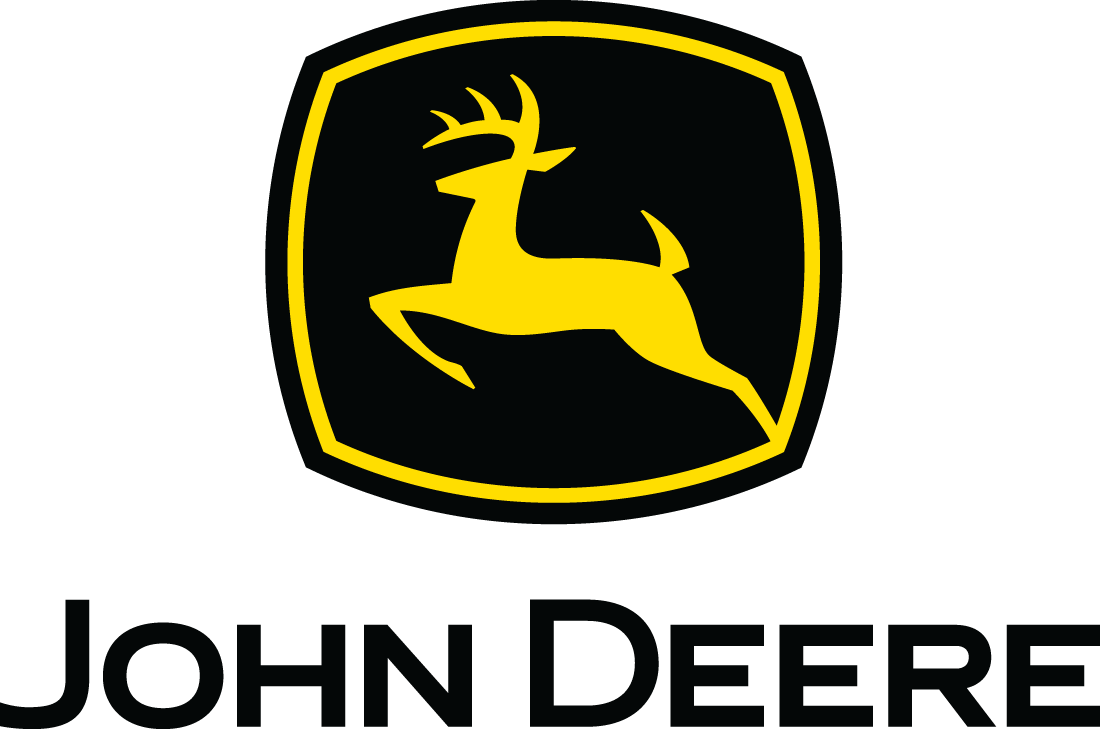
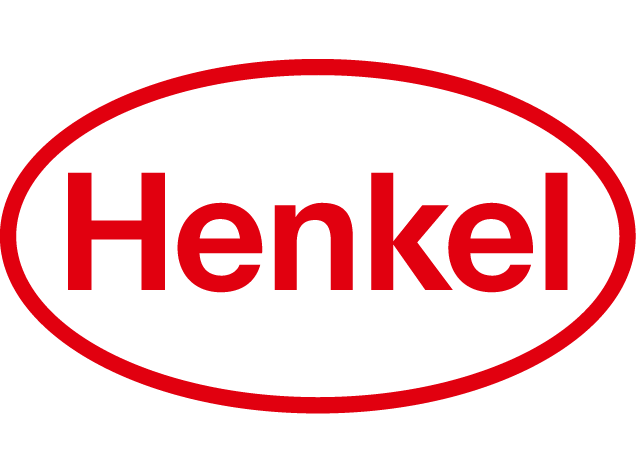
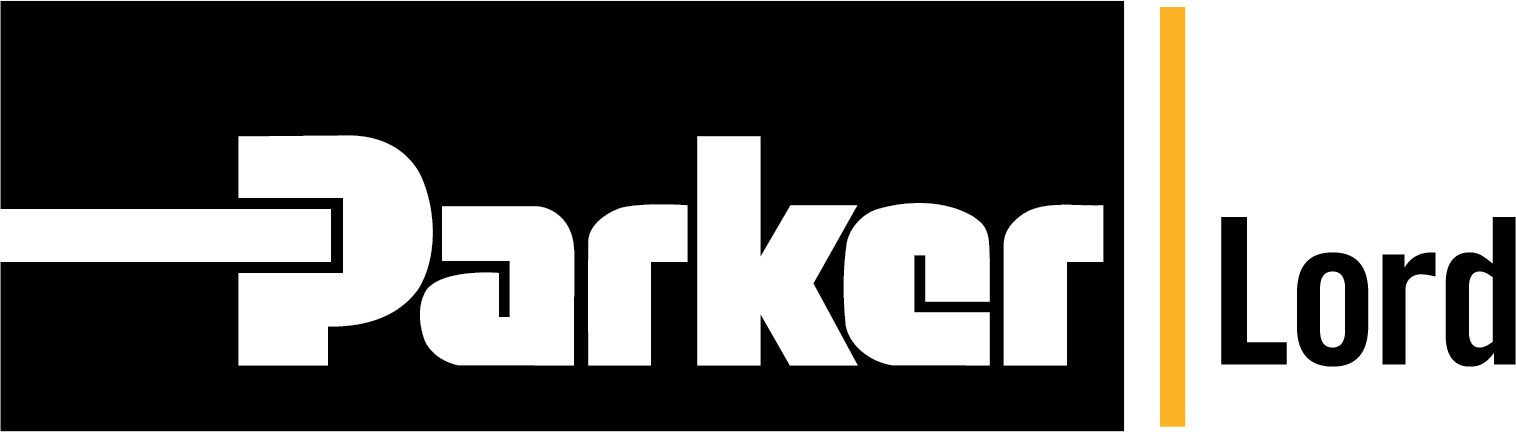
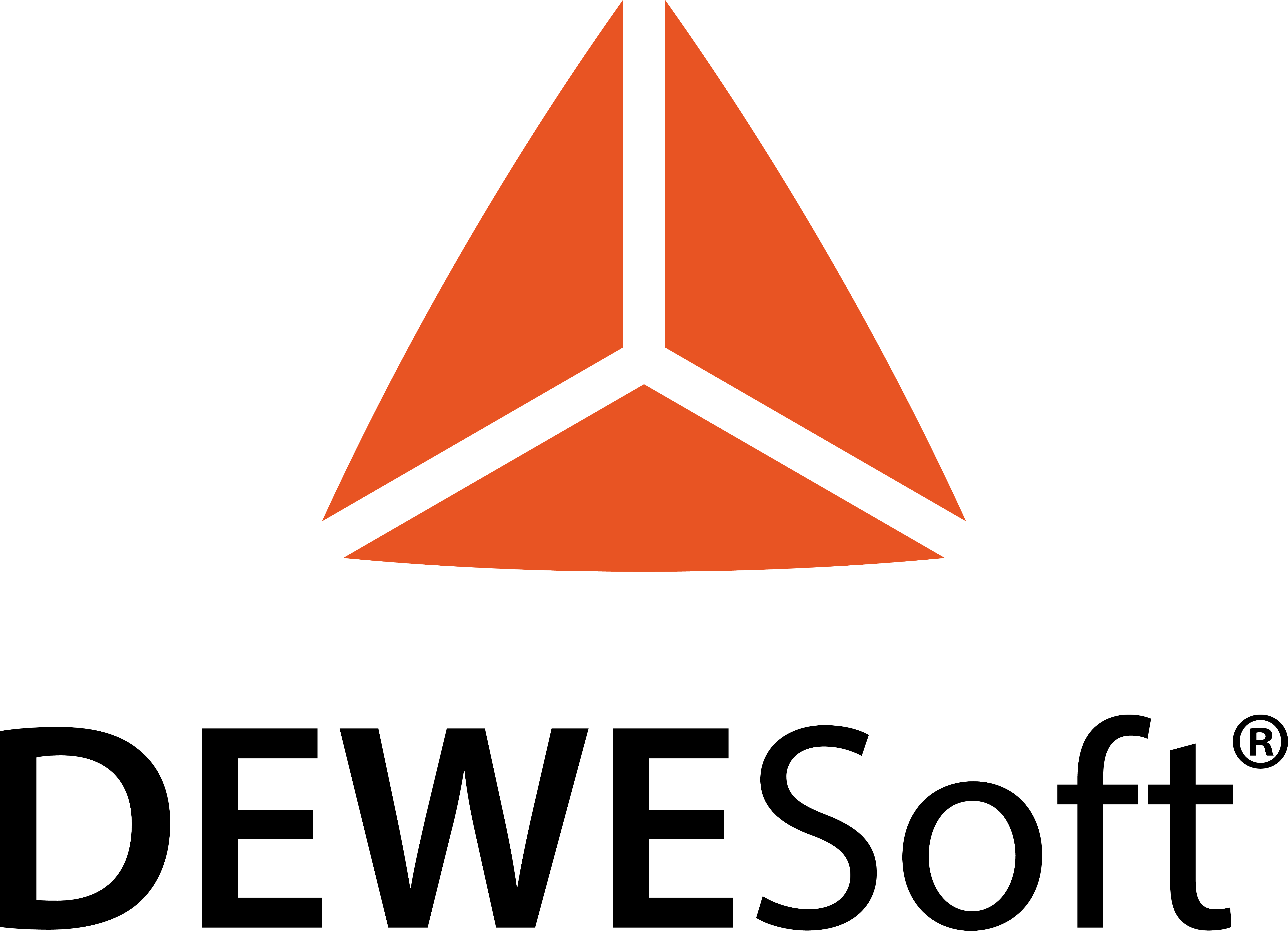
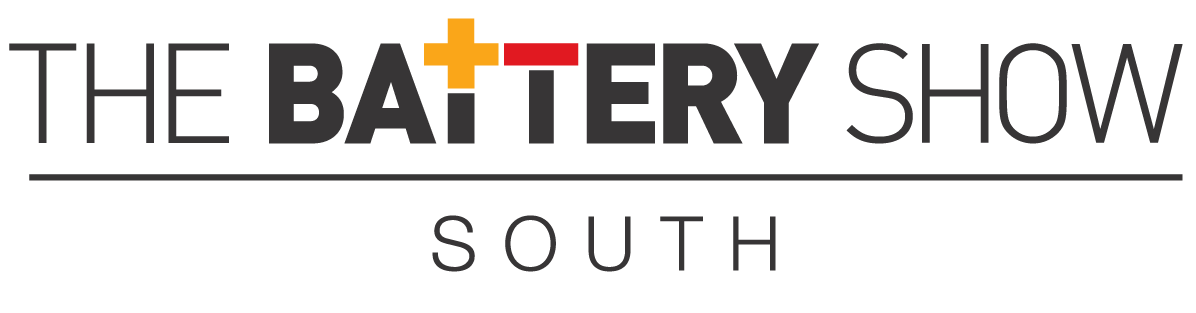
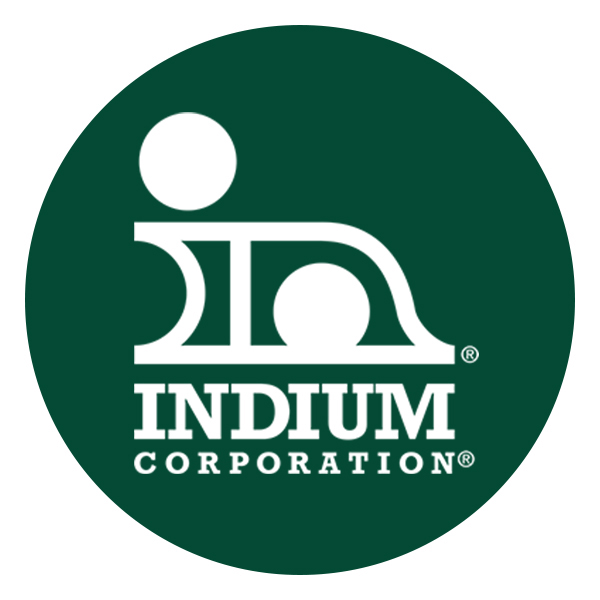
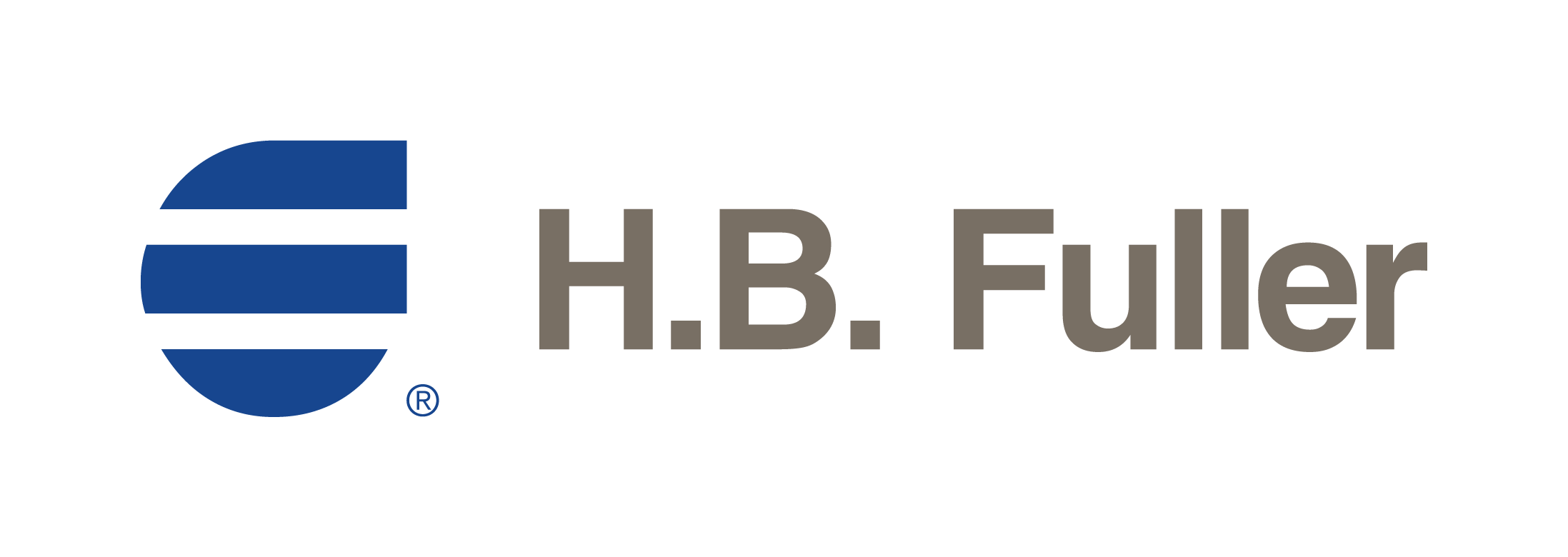