Battery welding
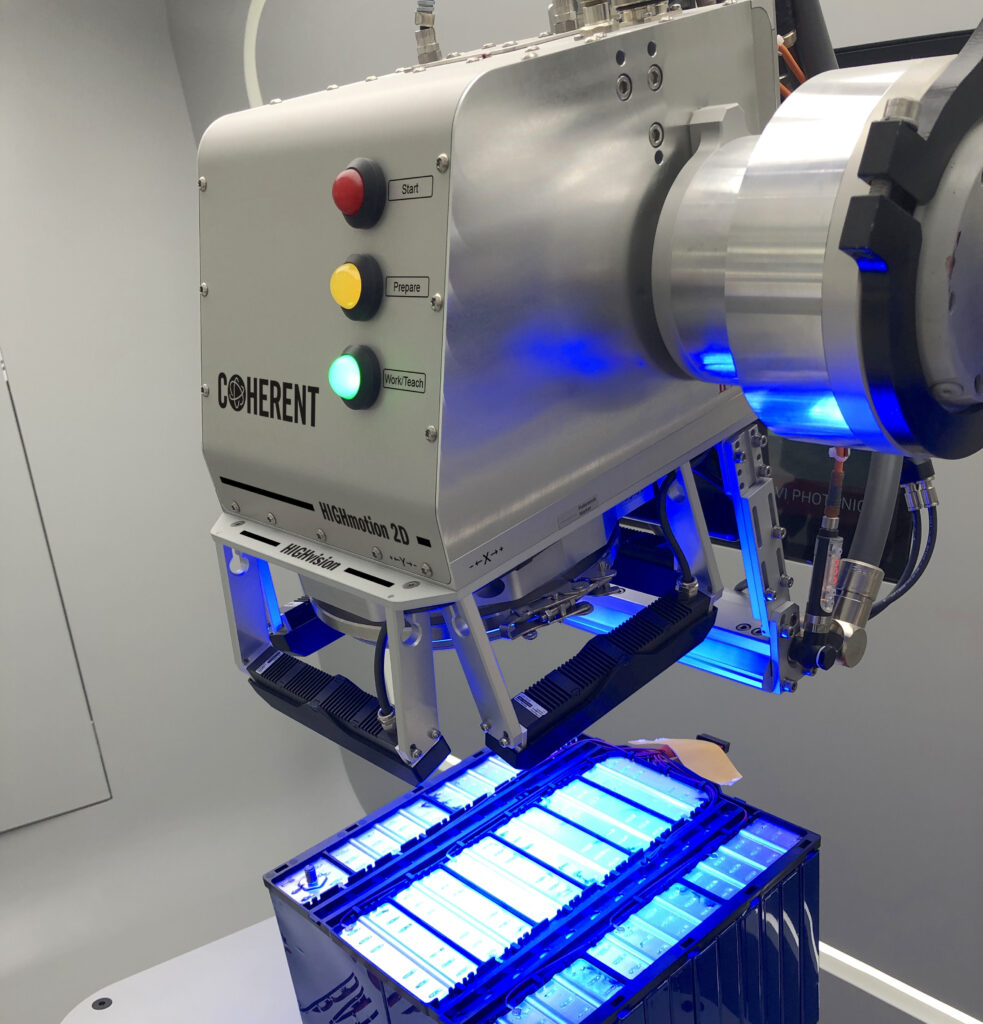
(Courtesy of Coherent)
United front
Welding experts give Peter Donaldson their views on how the technology is keeping abreast of developments in the EV batteries industry
Welding is a vitally important family of joining techniques for EV battery systems. A large battery might need thousands of individual connections, joining the positive and negative terminals of cells together in combinations of parallel and series blocks to form modules and packs of the required voltage and capacity.
As production ramps up and battery packs have to be built in volume and at speed, automation is becoming increasingly important, and welding techniques have to be adapted to mass-production environments. We’ve quizzed industry experts for some insights into how the technologies are being applied and how they are evolving.
Mark Boyle at Amada Weld Tech says the market is particularly hot now. He says there is a growing demand for EVs and for energy storage at charging stations, developments being driven by pressure for higher capacity, longer range and faster charging, with further impetus from government regulations.
At laser welding specialist Coherent, Jeff Franks points to continued and very high demand for production capacity, with pressure for continuous improvement in yield and quality. “Many of our customers are constantly innovating, so we must innovate in step with them,” he says.
With electrification spreading to all manner of vehicles, boats and aircraft, production capacity is expanding in all areas to try to keep up with demand, according to Sebastian Holtkamper at Hesse Mechatronics. “The market is being pushed by a large number of heterogeneous participants, who bring a mix of many different requirements,” he says.
Smaller companies tend to use older techniques such as spot/resistance welding and skilled, experienced operators for special products in small batches and prototypes, notes Phil Carr at Carrs Welding. “As usual, the OEMs are leading the way with laser welding, and entire lines of single-mode machines are being installed to weld cells and busbar systems.”
There are only so many ways to join materials together, and for battery applications – particularly where high currents and voltages and tough operating environments are encountered – welding beats alternatives such as soldering, conductive adhesives and mechanical fasteners. Welding provides a robust, strong, and comparatively lightweight fusion joint, whereas soldering and conductive adhesives are prone to melting or weakness at high temperatures.
Also, mechanical fasteners carry a significant weight penalty, so they tend to be restricted to some dissimilar metal joints and to prototype battery systems. Further, welding provides significant cost advantages over conductive adhesives and fasteners, and is a much more repeatable, rapid and accurate process than soldering, Mark Lakis at Wiegel adds.
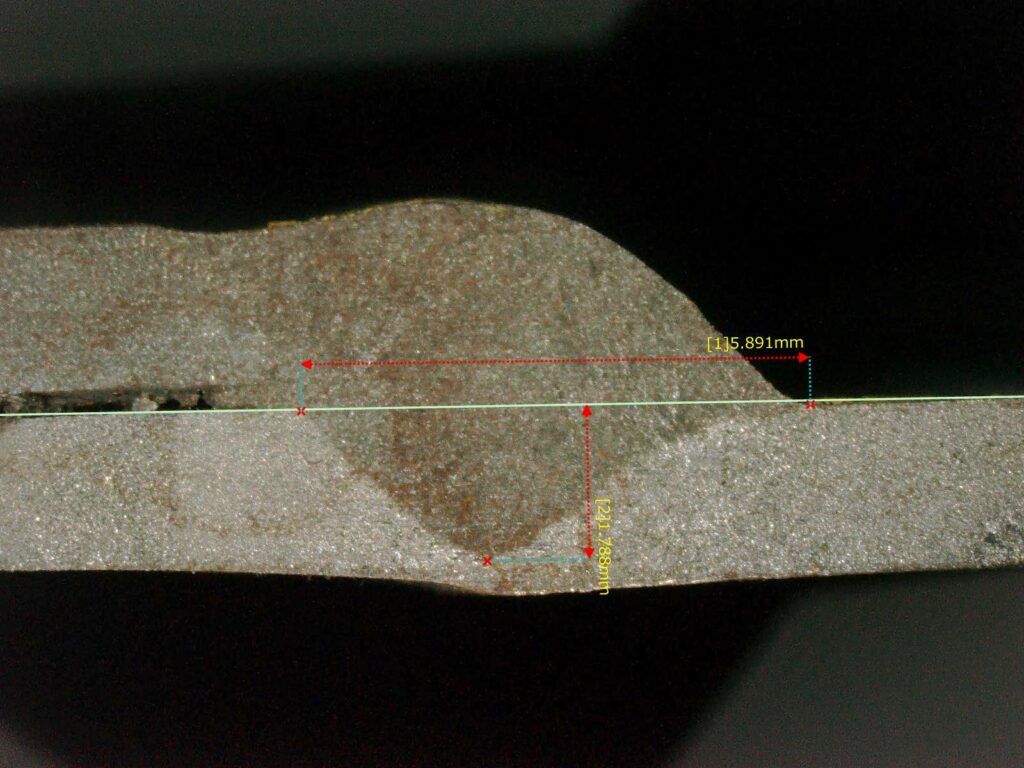
(Courtesy of Keyence)
Material issues
At the highest level, reliability and process speed are the main engineering challenges in welding battery contacts and structures, he notes. Carr concurs, and cites cost and customers’ desire to use the latest materials, such as grades of aluminium that are super-light but hard to weld and seal shut. “Copper seems to be more prevalent, as its cost has remained stable and its extra conductivity is a bonus because it reduces losses,” he says.
“We see a demand for highly controllable weld penetration, highest seam quality with little or no spatter as well as the highest throughput possible,” Jeff Franks at Coherent notes.
In general, Boyle says, manufacturers want light, highly conductive, increasingly efficient battery packs, and they often choose materials such as copper and aluminium, both of which are difficult to weld, making joining current collectors to cell cans a challenge.
“Depending on the battery design and materials, the metallurgy of the welds prevents some joints from being made with sufficient strength to handle the current,” he says.
“One of the more difficult combinations for any type of welding is steel and aluminium,” he explains. “Cylindrical cells are often made of cold-rolled, nickel-plated steel, to which manufacturers want to weld an aluminium tab because it is lightweight and very conductive.
“But steel and aluminium don’t play nicely together. The fusion process melts both materials, and as they re-solidify they form a brittle, intermetallic compound which typically falls apart under vibration or stress.”
Design for manufacture is another challenging area that Boyle points to, particularly the positioning of positive and negative terminals, which can be on the same or on opposite sides of the cell; top and bottom, for example.
“Terminals on opposing sides requires a complicated flip of the heavy battery pack to weld both sides,” he explains. “When terminals are on the same side, the current collection plate needs to be intricately designed to allow access to the battery cans, and the landing of the outer ring is often critical to achieving a good weld.”
Wide tolerances in manufacture also present problems, he adds, particularly of the cells themselves, as varying terminal heights and positions make automated welding more difficult.
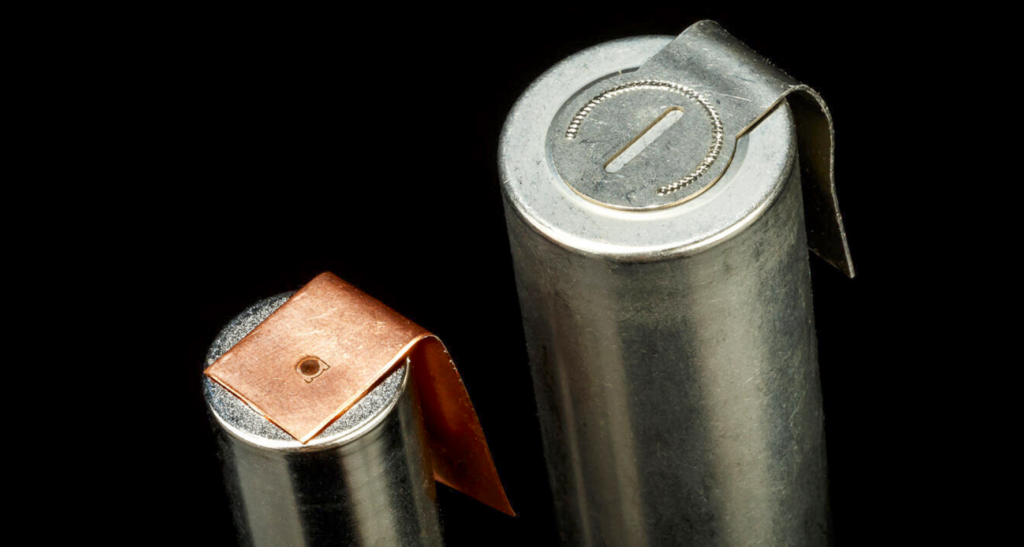
(Courtesy of Amada Weld Tech)
The need for speed
Boyle also notes that high demand usually translates into a need for high-speed production, which can limit the number of welding technologies considered or require large capital investment for multiple machines.
Resistance welding on a production line, for example, typically involves a pair of electrodes moving down onto the workpiece, making the weld, moving (indexing) up, along and down again in a cycle that repeats roughly every second.
“However if you use a laser, particularly with a scanning head, it can scan through a range of eight or nine cells, depending on the pack, cell size and field size of the scanning head, in about the same time and then index to the next group of cells,” he says.
He adds that laser welding is a little more complicated because it is a non-contact process that doesn’t physically push the workpieces together, yet requires intimate contact in order to make the weld. That makes tooling and fixtures a necessity, so a balance has to be struck.
“If high-speed production is not the be-all and end-all for the customer, they opt for resistance welding because it provides that contact force, reliability and repeatability,” he says. “If high speed is required, then companies are going to laser.
“Each company has to weigh the pros and cons. A laser requires a larger initial investment, whereas resistance welding equipment costs less but uses consumable electrodes. And if you do need speed, you will be bulk-buying multiple resistance welding machines.”
Challenges facing engineers can be very different, depending on their individual background experience and specialisation, according to Holtkamper. “However, what they have in common is the need to keep an eye on multiple welding technologies. Laser, ultrasonic smart welding, wire bonding and others can be applied to most typical products, but only a deep evaluation of the alternatives helps to uncover big advantages for particular products or product variants,” he says.
The alternatives differ in their process requirements, and affect the choice of connector materials. For ultrasonic smart welding, copper alloys of a particular hardness are favourable for certain processes, Holtkamper says, while the clearance for top access for the welding tool must be provided and cleanliness ensured.
For laser welding, however, surface requirements and maintaining zero gap between components to be joined are much more important. And as a fusion process, material combinations and safety issues have to be considered in design and production.
Engineers are also increasingly faced with tight timelines to the start of production (SOP), Holtkamper adds. “The timelines to SOPs are shorter than ever, and collide with the long lead times of high-performance equipment,” he says. “In these situations, cooperative development and reliable relationships are of high value.”
While there many kinds of welding, in EV battery applications the most common are resistance welding and laser welding, along with ultrasonic welding and wire bonding, and benefit from standardisation for mass production. However, these techniques differ in terms of speed, creation of unwanted intermetallic compounds and process controllability, according to Holtkamper. Outliers include micro-TIG welding, ultrasonic-assisted laser welding and electron beam welding.
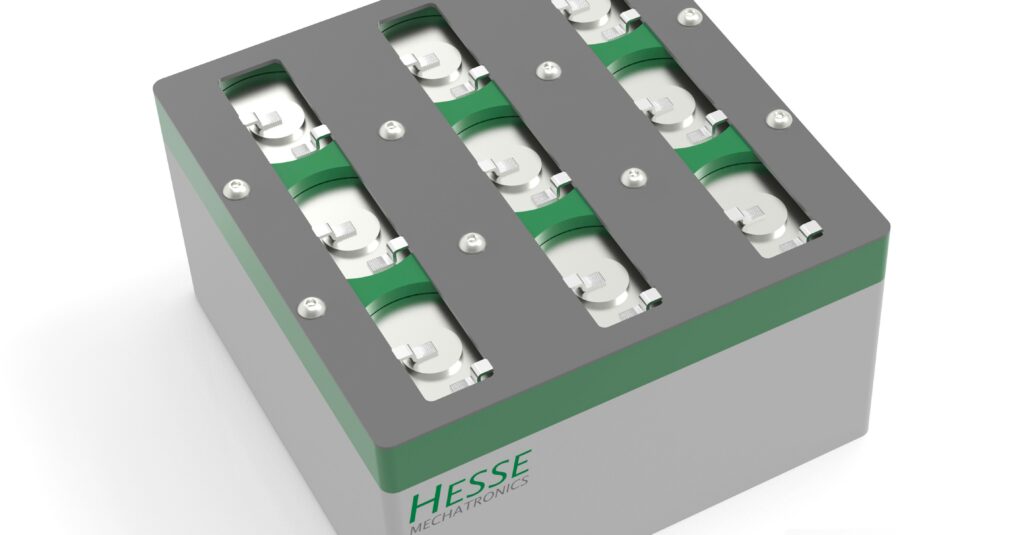
(Courtesy of Hesse Mechatronics)
Resistance is useful
Resistance welding passes an electric current between a pair of electrodes and though the materials to be joined, relying on the heat generated by ohmic resistance to melt and fuse them. It is a proven and relatively cheap process, but has limitations in the applications and geometries to which it is suited.
As a process that relies on physical contact, it requires the battery design to allow good access. It is not suited to seam welds, it is relatively slow – as explained above – and electrodes need to be maintained and/or replaced, and cannot easily handle conductive materials and increasing material thicknesses.
The problem with highly conductive materials stems from the process’ reliance on resistance to generate heat. Contacts need to be highly conductive in service, so a balance has to be struck between conductivity and weldability of the joint.
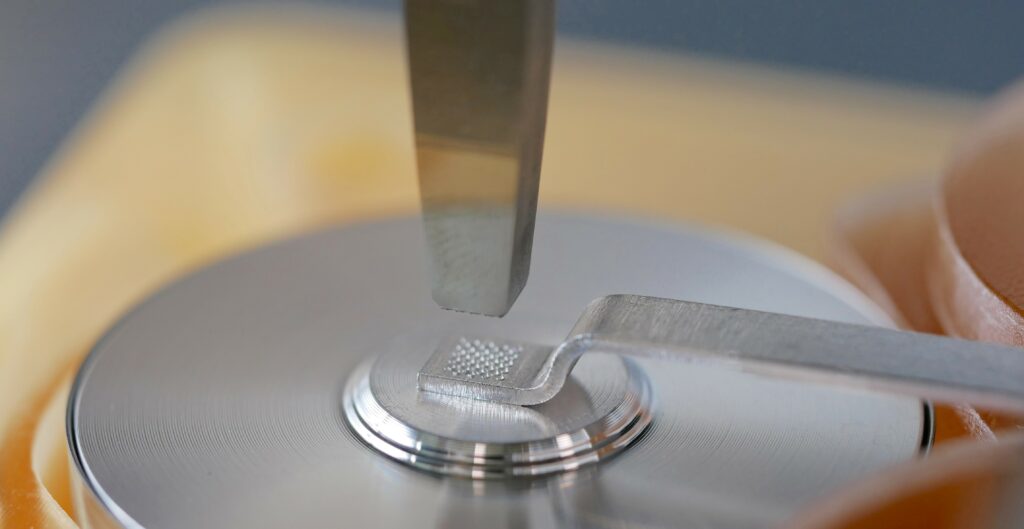
(Courtesy of Hesse Mechatronics)
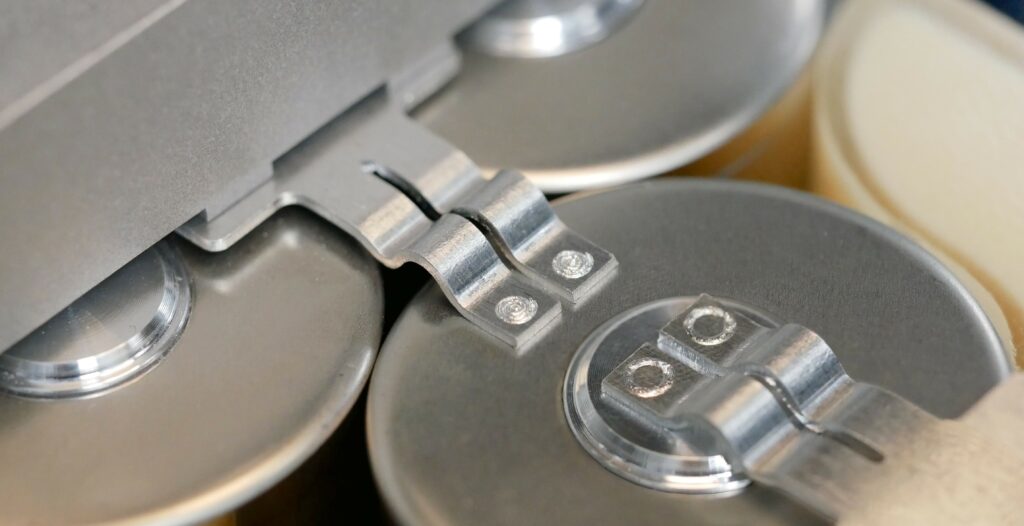
(Courtesy of Hesse Mechatronics)
Light fantastic
Laser welding has become the industry’s preferred option for speed and flexibility, notes Carr. Much of that flexibility comes from the ability to reposition the beam rapidly, using technology such as ‘galvo’ scanning heads, which use tiny electromagnetic actuators to angle beam-steering mirrors, Boyle says.
Quantifying welding times for laser techniques, Holtkamper says they can be as short as a few milliseconds per cell. Modern systems also come with standardised clamp-and-weld functionality to hold the work as the joint is formed, improving repeatability, he adds, and can shape the beam to enable both spot and seam welding. Connections formed by lasers exhibit low electrical resistance, and the area around the weld affected by heat is small.
While the high capital cost of laser welding is coming down, the process still has a number of other drawbacks. In addition to the extra complexity brought by the need for tooling to hold the work in place, it can be difficult to control the formation of the intermetallic compounds, such as aluminium-copper and aluminium-iron.
However, this is true for all fusion welding processes, not just laser welding, Boyle points out. Further, capital costs grow with laser power, which also mandates more laser safety measures, and there is a need as well to control residues and splatter, and to minimise the risk of penetrating the cell, he adds.
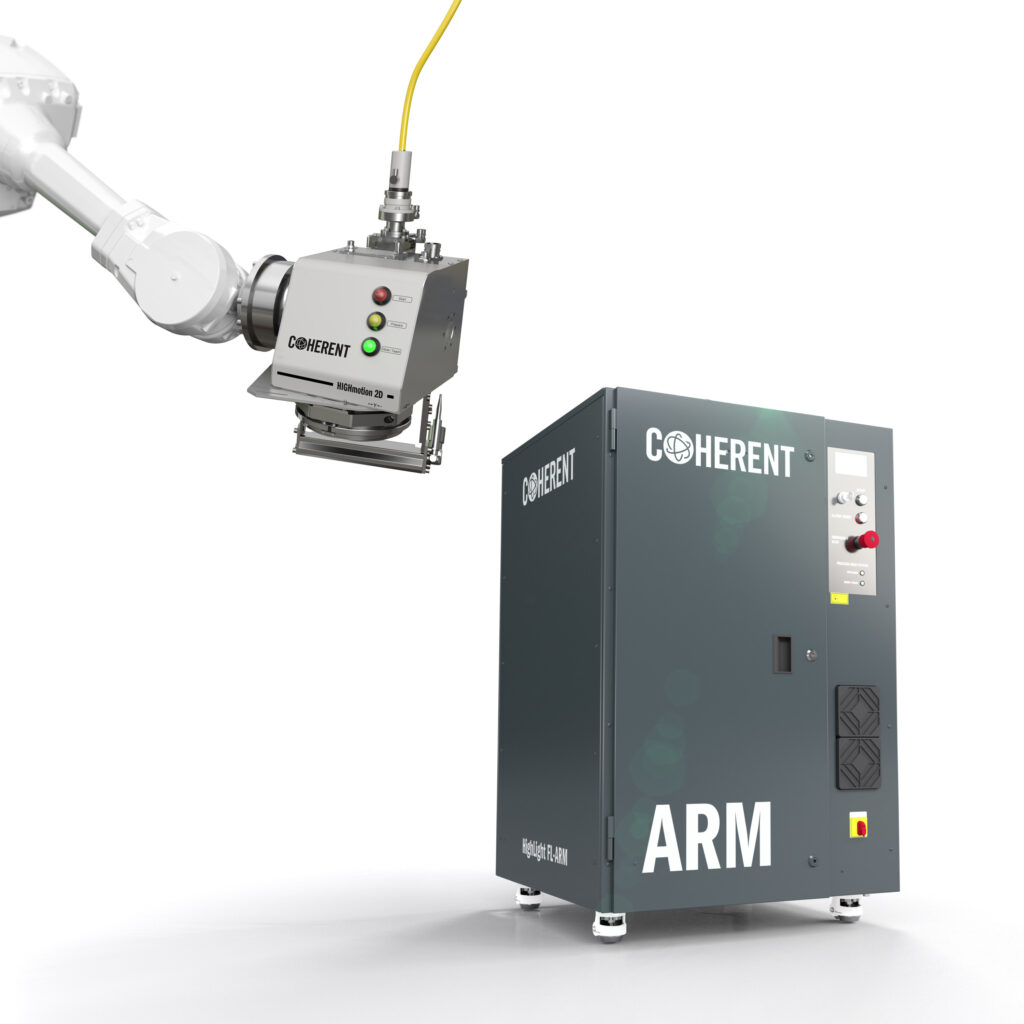
(Courtesy of Coherent)
Sound joints
Ultrasonic welding (UW) uses the combination of pressure and ultrasonic vibration that, in metals, causes high-pressure dispersion of surface oxides. In turn, this brings clean surfaces into very close contact under high pressure and at localised temperatures, which while high are below the melting point of the metals.
Under these conditions, metal surfaces diffuse into each other to form diffusion bonds. As the metals don’t melt, UW does not make fusion joints.
According to Holtkamper, UW principles are well-known, standardised production equipment is available, the process is well-suited to materials with high thermal conductivity and offers good traceability. But although UW needs no elaborate support tooling, it does require a rigid underlying structure.
Wiegel recently showed that UW is also very effective for creating busbars of composite thickness (meaning the thickness varies along and across the bar), having been awarded a patent for it earlier this year. The process involves cutting out a base component and a thinner foil sheet, loading both into a fixture according to how they are to be joined, then passing the fixture holding them through a UW machine and subsequently trimming away excess material to produce the final part.
Compared with alternative methods such as laser welding and brazing, using UW to produce composite thickness busbars for battery current collectors produces wider and stronger welds of greater cross-sectional area, the company says.
Mark Boyle notes that UW handles dissimilar metals well, but the vibration can damage nearby components. The process also produces fine dust that has to be removed from the battery, or prevented from entering it.
Carr points out that, in battery applications, the process is only used on non-ferrous metals and is limited to joining interconnects and busbars. Holtkamper adds that ‘smart welding’ combines high welding power of conventional ultrasonic welding equipment with the flexibility, precision, speed and advanced process control of wire bonding machines.
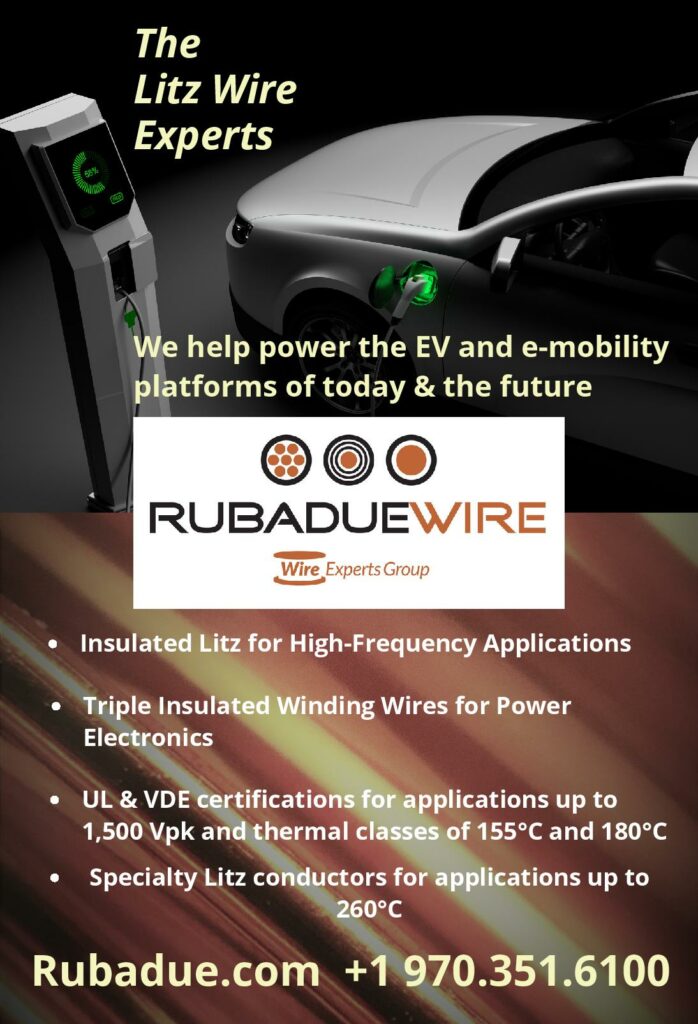
Wire bonding
Wire bonding can be described as an ultrasonic and compression process in which a combination of time, ultrasonic energy and pressure is used to create an intermetallic bond between the wire and the contacts. The wire is fed though the machine and the welding head to provide the connector material, and the tool on the head is called a wedge, hence the term ‘ultrasonic wedge bonding’.
The technology has been used in the semiconductor industry for decades, and is also familiar to the automotive industry and conforms to its standards, Holtkamper says. He adds that the looped form of the connection allows movement that absorbs stresses created by thermal expansion, that the process provides good control of electrical resistance, and that the wires can also act like fuses, parting to isolate faults.
Further, because the welding tool moves from one contact point to another, it is easy to reprogram it within its motion limits to adapt it to different products and contact configurations. Finally, any faulty wire bonds can easily be sheared off and in some cases remade, he says.
The main limitation of the process is in the dimensions of the wire it can handle. For round wire, the upper limit is between 500 and 600 µm in diameter, while for ribbon wire it is 2000 x 400 µm, a consequence of which is that contact areas are limited. Also, applications that use multiple wires to connect each cell to a current collector need relatively long welding times.
Micro-TIG welding is a version of the tungsten inert gas (TIB) electric process used for small parts made from non-ferrous metals. Enabled by advances in high-frequency power supplies that improve arc stability and control of small currents, it is very good for joining copper while offering what Amada Weld Tech describes as a fairly relaxed process window with respect to part fit-up and positioning tolerances of the electrode to the parts. Although relatively cheap to implement, it is a slow process and consumes electrodes.
In batteries, it offers a good solution for welding busbars that would otherwise need a brazing material for resistance welding, or a high-power laser welder, according to Amada. Butt, fillet and lap welds in copper are routinely achieved up to and a little beyond a thickness of 0.02 in, says the company, which stresses the importance of using the welding system’s pulsation function to avoid porosity in the weld.
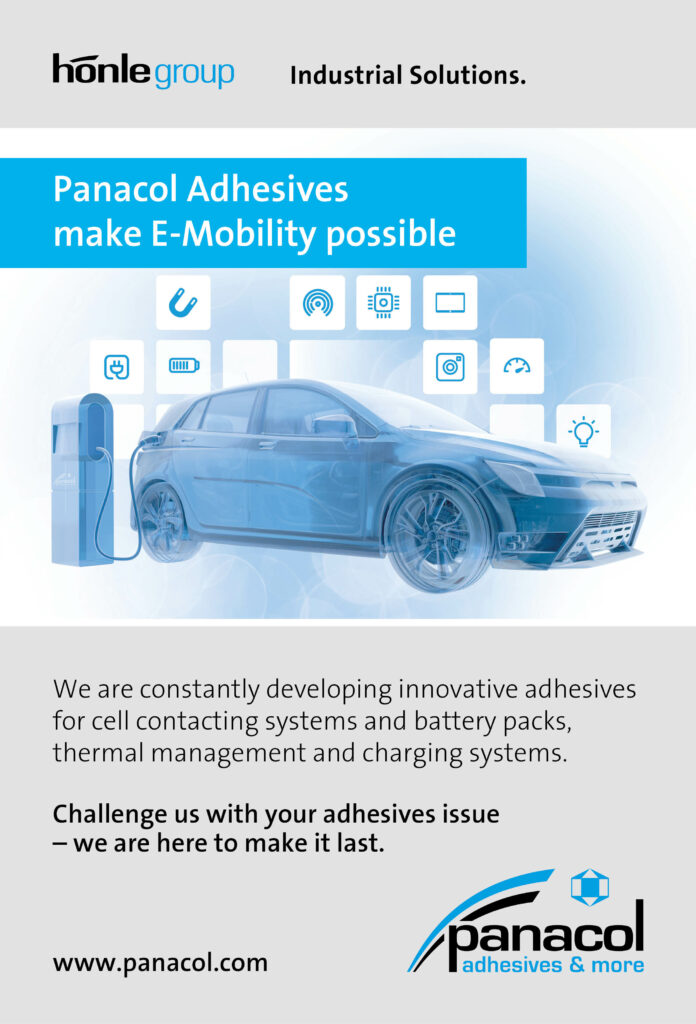
Advancing processes
Of these, laser and ultrasonic welding processes dominate in EV battery manufacture – with laser welding the preferred solution for mass production – and continue to be improved and refined.
“We see a lot of laser welding and ultrasonic wedge bonding for the larger packs,” says Boyle at Amada Weld Tech. “If the packs or the overall volume are smaller, then resistance welding is often used. Micro-TIG comes up for specialised battery packs with low-volume production.
“Recent developments in galvo scanning, beam steering and process monitoring allow for faster speeds and improved process error detection, respectively,” he adds. “We have added both galvo scanning welding and process monitoring to our systems for battery pack welders. In addition, we have used lasers of more and more power.”
Phil Carr notes also that advances in laser welding have reduced heat input into battery cells, enabling small spot sizes without sacrificing strength. He also points to the emergence of green and blue lasers, which work well on non-ferrous materials such as copper. “I can see them becoming more dominant, but the small spot of a scanning fibre laser is my preferred option for the next 5 years,” he says
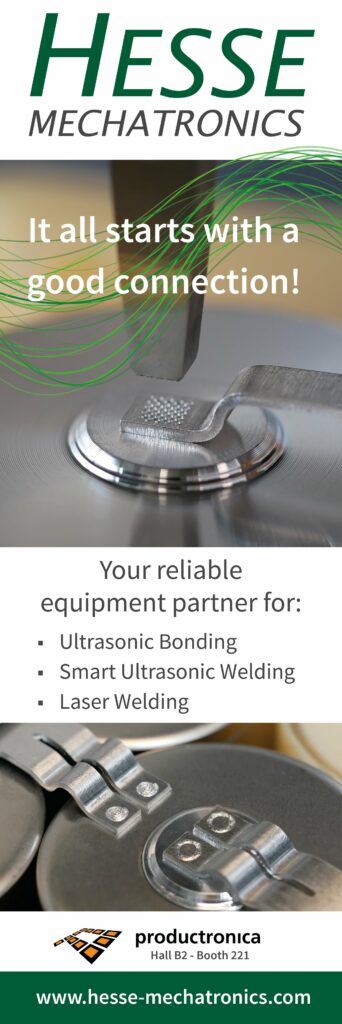
When the welds required are long seams that have to go around corners, for example when sealing the lids on the cans of prismatic cells, conventional solutions such as moving the laser head or the work along a gantry, or using scanning mirrors to direct the beam run into problems. A typical prismatic cell is about 20 mm wide by 300 mm long, and needs a continuous weld around the entire perimeter to provide a hermetic seal, even if the parts fit is less than perfect, and the durability to survive shock and vibration though years of service.
To achieve that, the weld needs good penetration, minimal porosity and no spattering, as droplets of metal inside the cell casing can cause short-circuits, while it must also keep the resulting heat stress on the cell.
Fibre lasers (FL) do all of this well, but Coherent points out that gantry systems are slow, while conventional optically scanned systems can suffer from weld variability as the spot changes from a disc to an oval shape of larger area as the beam angle shifts from the vertical towards either end of its scan travel, despite the use of lenses designed to minimise the unwanted effect.
Coherent’s solution to this is its Fiber Laser – Adjustable Ring Mode (FL-ARM) technology. In a FL-ARM system, such as the company’s HighLight ARM, for example, the beam has an additional outer ring of laser light that is concentric with the spot, and the laser power delivered to them can be varied independently using active closed-loop control to keep the energy that goes into the work much more consistent along the whole weld.
As well as spot elongation, FL-ARM can also compensate for changes in scanning speed, such as when the beam is steered into and out of a corner. Weld penetration depth and seam can also be controlled independently so that there is no longer a need to maintain tight tolerances in parts fit, says the company, which reduces manufacturing costs.
Other benefits include shrinking the heat-affected zone, higher scanning speeds and larger scanning fields. “Even highly reflective materials such as welding of copper see clear improvements with the technology,” Franks adds.
Independently of the welding techniques applied, welding machines are also getting smarter and more standardised, Hesse Mechatronics’ Holtkamper says, incorporating features drawn from mass-production technologies used in other industries. “For instance, pattern recognition can be freely programmed to find the right positions on batteries, BMSs or busbars in less than 10 ms,” he says.
“Another improvement that comes with standardisation is the traceability and monitoring of quality in real time,” he adds. “That means the data created during the process can be sent to manufacturing execution systems to judge the quality of the welds.” He further explains that quality systems also operate in a decentralised manner, with sensors and algorithms on the machines, enabling them to react in real time to changing conditions.
Hesse Mechatronics has launched a new laser welder designed to join thicker materials with larger contact areas, he adds. “Clamping and welding a contact in one shot will serve the high demand for flexibility without needing costly consumables or tooling,” he says. “This is especially true for cylindrical cells.”
Architectural adaptation
Welding technology also has to adapt to novel battery architectures such as cell-to-pack and cell-to-chassis, particularly when it comes to automation. These architectures have been tending towards more conductive and thicker materials, especially in busbars, pushing solutions towards laser welding, Boyle comments.
“In general, moving away from modules/packs means some reduction in the number of welds in a pack or an EV,” Franks adds. “But as we keep seeing new applications this is having a negligible impact on the high demand we see for laser welding equipment.”
Holtkamper focuses on the higher level of integration these architectures represent, noting that it means the products to be processed can be larger.
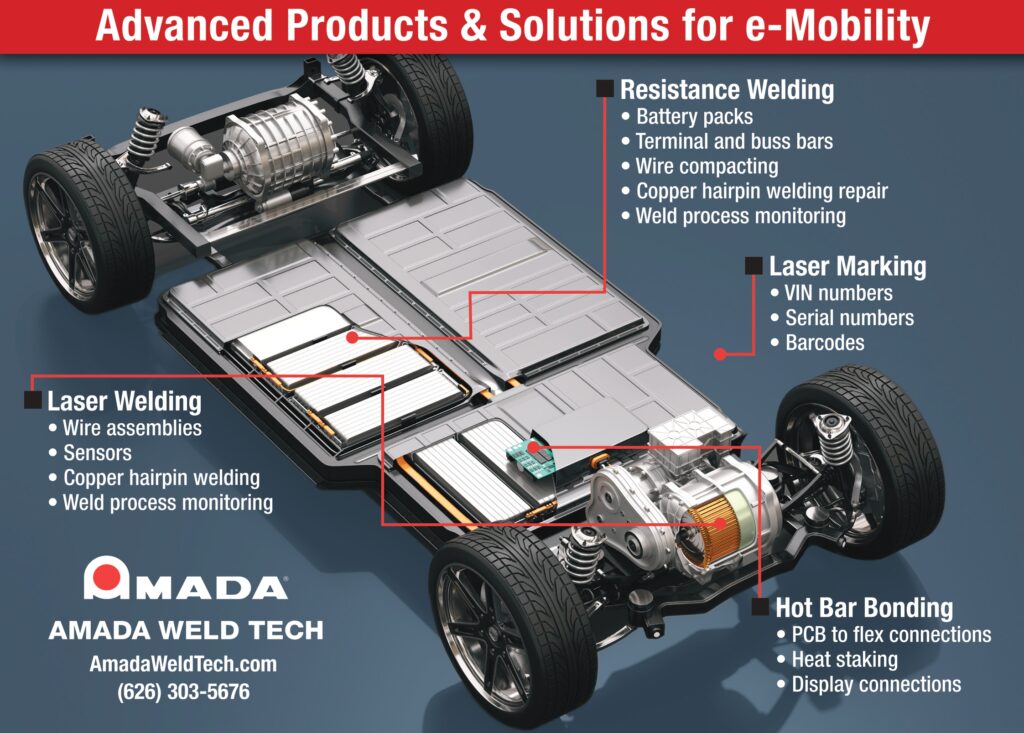
Emerging processes
Ultrasonic-assisted laser welding is a new process that is still at the r&d stage, Carr notes, and Carrs Welding is working with Brunel University to investigate the technology through the SoniLaser project.
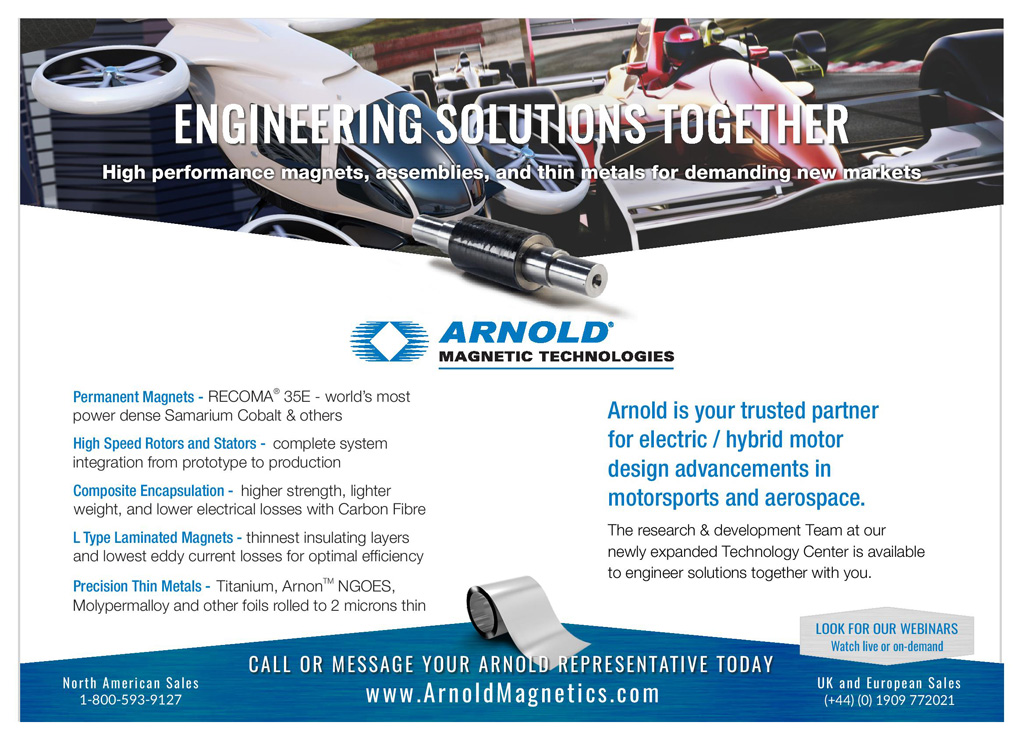
The idea is that the ultrasonic excitation will interact with the molten pool flow, the solidification mode, and the mixing of alloying elements and diffusion that happen during solidification. By breaking continuous flows such as Marangoni convection within the weld pool, the ultrasound will minimise the formation of intermetallic compounds and precipitates that can contaminate and weaken welds between dissimilar metals.
Another process under investigation for future application to EV batteries is electron beam (EB) welding, which works by focusing and directing a stream of electrons generated by an electron gun using magnetic fields, much as cathode ray tube TVs did. The electrons’ kinetic energy is converted into heat by impact with the workpieces, causing localised melting and bonding.
The technology is well-established in industry and is routinely used to join turbocharger wheels to shafts, for example. EB welding produces clean, high-quality welds with fine control of penetration depth and a very small heat-affected zone.
It is also potentially 20 times faster than laser welding. However, it has to take place in a vacuum, because any gas between the electron source and the work will scatter the beam, which is a problem for batteries as exposure to vacuum can damage cells.
Recently, a means of enclosing the electron gun in a vacuum box sealed to one side of the material to be welded has been invented, making it no longer necessary to put the whole battery module or pack in a vacuum chamber. The UK government’s UKRI is sponsoring a demonstration of the technology by a consortium of Aquasium Technology, Delta Cosworth and TWI.
While there are no completely new techniques applied to batteries, adaptation, combination and increasingly advanced automation keep them relevant.
ONLINE PARTNERS
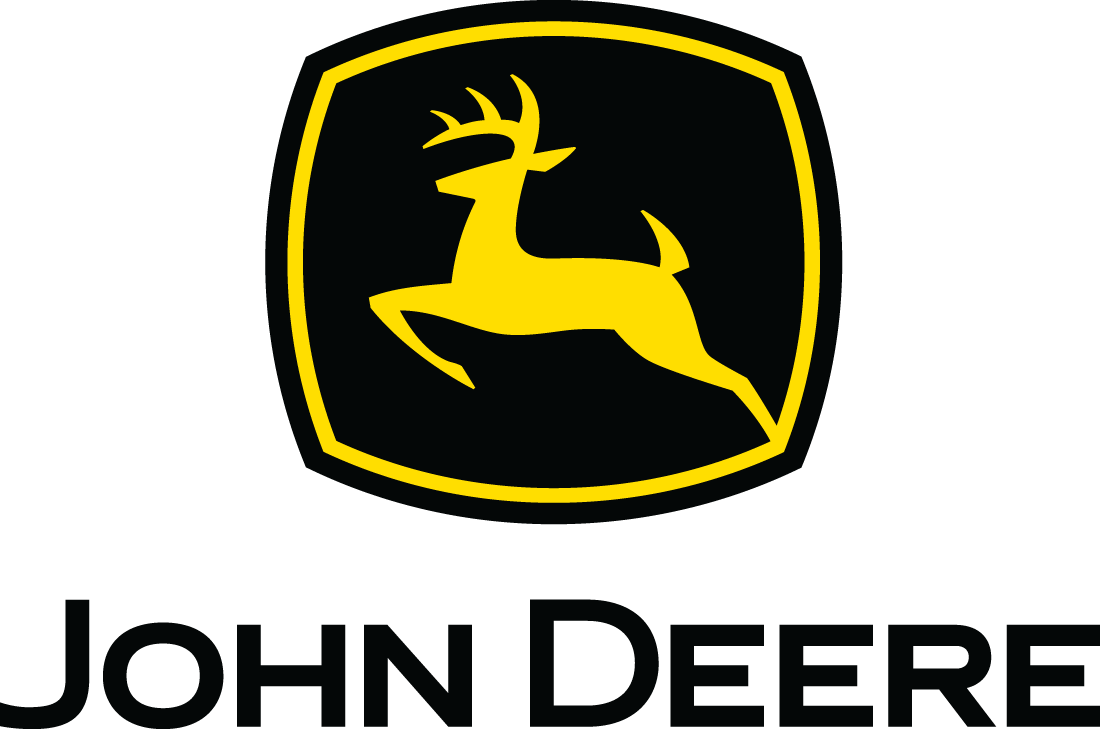
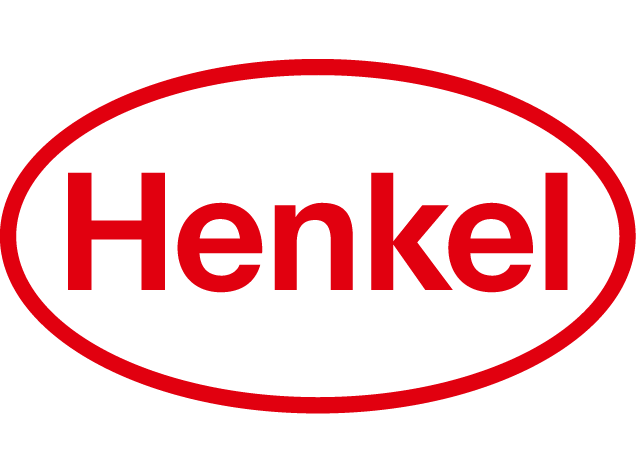
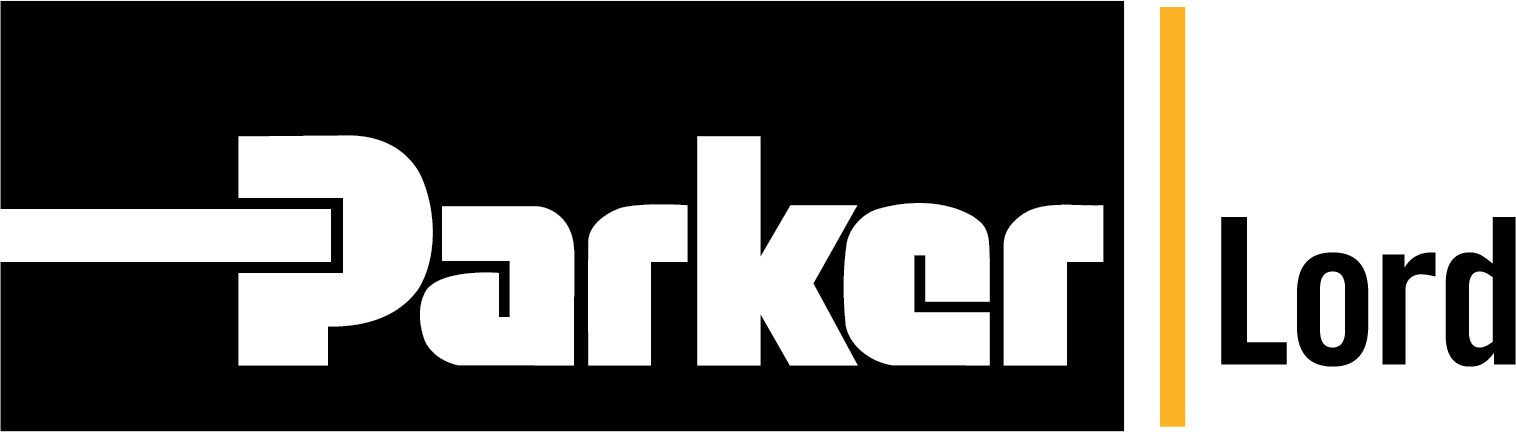
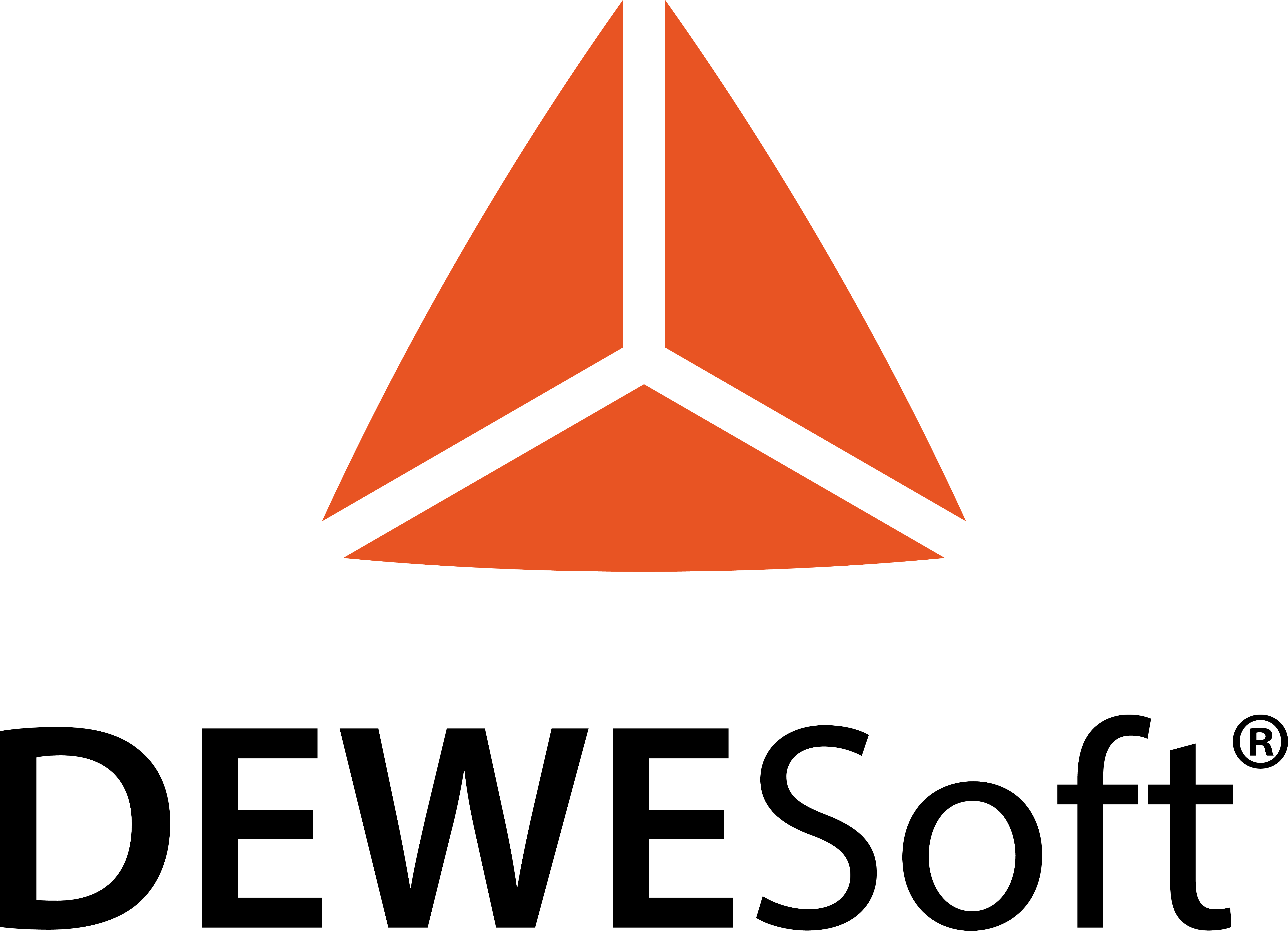
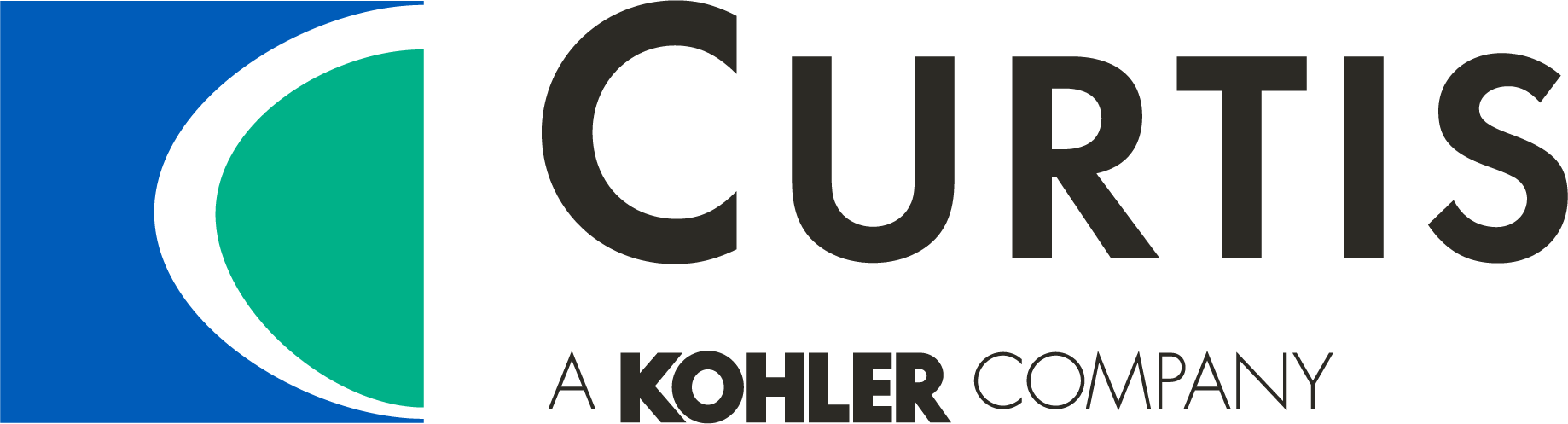
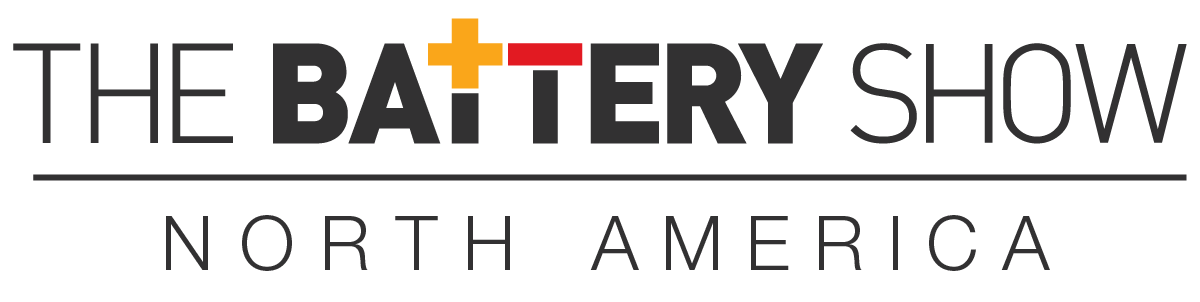